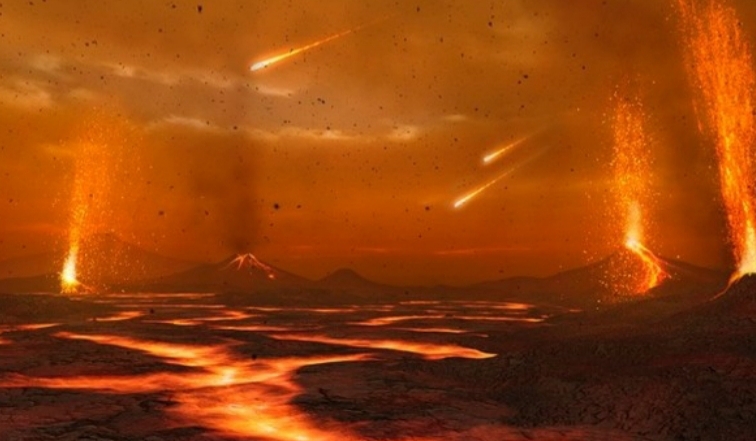
Could physics explain how life emerged on early Earth? © Getty Images.
There is a new theory about how life first emerged from the primordial goo, with physics enjoying a starring role.
From the expansion of the Universe to the motions of the tiniest subatomic particles, modern-day physics can help us interpret a dizzying number of natural phenomena. But can it explain perhaps the biggest mystery of them all: how did life as we know it begin?
Dr. Jeremy England, assistant professor of physics at the Massachusetts Institute of Technology (MIT), thinks “I was always interested in how the physics of big, messy assemblies of particles becomes life-like, ever since I was doing research on protein folding as an undergraduate,” England says. “It was the way I could successfully refuse to choose between theoretical physics and biology, which both were fascinating to me.”
England’s work is based on the well-established physics of thermodynamics – the science that describes how heat moves from place to place and is crucial for many natural processes. He calls his theory ‘dissipative adaptation’, as it aims to describe how structures emerge and change through the dissipation of energy, primarily heat, into their environment.
This process increases the entropy (the amount of disorder) in the surroundings, which Austrian quantum physicist Erwin Schrödinger identified as necessary for living organisms to function. Crucially, the increase in entropy makes it possible for the evolving structures to stay in what is known as a ‘non-equilibrium state’.
Usually a system (which could mean anything from a box of gas to a complex structure) comes into equilibrium with its environment. This means that there is no net flow of heat between the system and its surroundings. For example, if you leave a cup of hot tea on the table, it will eventually reach the same temperature as the room, much to the chagrin of the tea-lover who was looking forward to a cuppa.
But living things are in a non-equilibrium state, taking energy from sources such as sunlight and food and pushing that energy out – ‘dissipating’ it – into their surroundings. This enables a living organism to reduce its own entropy, so it can grow and build structure.
And it is the physics of such non-equilibrium states that England and his team investigate, by using computer simulations to look for situations where life-like behaviors emerge spontaneously.
Beyond biology
This is not the first time that a physicist has attempted to take on the deeper questions of biology. In 1944, Schrödinger published a book based on a series of lectures he had given in his adopted home of Dublin. The book, What Is Life?
emphasized the central significance of energy flows and entropy. In the book, he also suggested that biological inheritance would depend on what he called an ‘aperiodic crystal’ – a molecule that could carry information in its structure – a prediction that was fulfilled with the discovery of the structure of DNA.
“The beginning point of my current line of research – which really has veered away from biology, at this point – was realizing that to think about the physics, you have to take what’s interesting about life and break it into separate well-defined physical phenomena that you can then talk about in terms of thermodynamics. So, for example, living things make copies of themselves but not all self-replicators are alive,” says England.
The behaviors that England is targeting include reproduction, harvesting energy, natural selection, and the ability to anticipate the future.
Snowflakes are complicated, forming due to liquid water crystallizing into ice, but they are not alive © Getty Images © Getty Images
Sometimes these effects can be seen in simple, familiar phenomena that bear no resemblance whatsoever to life, such as snowflakes and sand dunes, he says. But in both cases, these structures are able to form as a result of releasing energy into the surroundings.
“In the case of a snowflake, it’s the heat released by the exothermic [heat-emitting] crystallization of liquid water into solid ice,” he says. “In the case of a sand dune, the flowing air gets the sand grains moving, but then they stop again because they rattle against each other and lose that energy as heat to the surrounding air.”
Entropy and evolution
The evolutionary history of an organism is stored in its DNA, molding its current form. England believes that an organism’s history of dissipating heat and therefore increasing entropy also help shape its structure. Without DNA to act as a record of changes, England believes that the physical form of the structures can hold information.
“Think about a glass being sung at by an opera singer, and it resonates and violently changes its shape and shatters. Once it shatters, it is much worse at absorbing energy from the song, as it is a pile of shards. It changes its shape much less, it has become much more stable,” he says.
“But those shards are not a random arrangement of glass, they contain a lot of information about the shape the glass was in when it shattered. So even though they are bad at absorbing energy, they have a signature of a moment in history when the opposite was the case, which can be reconstructed with the right detective work.”
Sometimes, in the team’s simulations, a surprising level of self-organization emerges. For example, when they begin with a virtual soup of different simulated chemicals interacting, some start to take over at the expense of others. They then begin to dominate as they prove better at harvesting the available energy.
“There are lots of things we think of life being distinctively good at, for example energy harvesting, predictive computation [anticipating the future] and self-repair, that we may be able to get to self-organize even in the absence of Darwinian evolution by self-replication and natural selection,” England says.
Though still in the early stages, the theory is not without its critics, and England himself agrees that there is a huge gap between simply observing life-like behavior and life itself.
“All life we see is the product of countless competing of past generations of things that were already alive and got to co-evolve for a long time,” he says.
“Also, any living thing is a composite of many distinctively life-like behaviors, and they do not necessarily all come hand-in-hand at the outset. For example, something could be a very good energy harvester and not necessarily be in any way capable of self-replication, and I do not claim to know anything about how the package deal we call life first gets bundled together.”
What he does believe, however, is that his team’s work is enriching what he calls the “starting toolbox” for life to form. For the moment, their work is purely based on computer simulations, though other researchers are starting to take up the idea and work on investigating similar thermodynamic effects in physical experiments.
We may not have the answer to how life began yet, but dissipative adaptation gives us a clearer picture of one of the fundamental principles that has encouraged the emergence of living things.
This computer simulation by Jeremy England shows particles in a gooey fluid. The turquoise particles are being driven by an oscillating force, which leads to bonds forming over time © Jeremy England
Source: Brian Clegg / Science Focus
Comments are closed.